Introduction
There exists an international scientific consensus that existing genetically engineered foods are at least as safe as their closest corresponding non-GMO counterparts [1]. This consensus is drawn on decades of research and thousands of studies. Despite this fact, there still exists a broad gap between the science and public perception of the topic. According to PEW reports, this gap is wider than on any other topic for which a strong scientific consensus exists [2].
This is significant because we know several other scientific topics have remained highly controversial in the court of public opinion for decades after having achieved mainstream acceptance among experts. Young Earth Creationists have been trying out various strategies for roughly a century to undermine the teaching of evolution in public schools in the US [3]. Rejection of Anthropogenic Climate change is ubiquitous in the US, and even extends to the POTUS himself, despite the weight of the evidence and resulting scientific consensus to the contrary [4],[5],[6],[7]. Although its prevalence has waxed and waned over time, vaccine opposition has been a near constant presence ever since the discovery of the cowpox vaccine [8],[9]. So, for there to exist an even bigger gap between science and public perception on GE foods than for any of these other topics, is no trivial matter.
One of the most common reasons given for trepidation with respect to GE foods is the idea that it’s a more radical way of altering our foods than more traditional methods of artificial selection, and that we therefore can’t know whether or not GE foods are bad until they’ve been around for several more human generations. This view relies on an argument from ignorance logical fallacy, and presents a double standard with no biologically plausible justification. One fact that proponents of this view rarely acknowledge is that it also leads to the generation of testable hypotheses, the results of which actually suggest the exact opposite. Allow me to explain.
The Scientific Consensus
In previous posts, I’ve pointed out what we mean when we speak of the international scientific consensus on GE food safety consists of two major claims:
- All currently approved GE crops have been tested on a case-by-case basis and the weight of the evidence suggests they are at least as safe as their closest non-GE counterparts.
- Nothing about the process makes unpredicted dangers any more intrinsically likely with modern molecular GE techniques than other methods of altering an organism’s genome.
I’ve already outlined the evidence for the first point in previous posts [1]. However, the second point directly bears upon the aforementioned double standards and arguments from ignorance comprising many anti-GMO fears and talking points. The purpose of this article is to explain how we know this to be the case.
The Genetic Code is a Universal Language
Before delving into what I believe to be the most intelligently-formulated versions of this set of concerns over GMO food crops, I want to clear up some even more pervasive perceptions that are based almost exclusively on malformed understandings of basic genetics and the evolutionary relationships between species. See, most applications of transgenesis involve the insertion of no more than one to three new genes. And although it is generally well-accepted that humans have been altering our food for thousands of years, those who are distrustful of biotechnology often argue that that doesn’t count on the grounds that cross-breeding doesn’t entail the insertion of a gene from a totally different species, phyla, or even kingdom of life. The argument usually takes the form of “selective cross-breeding is not remotely the same as putting a fish gene into a tomato!”
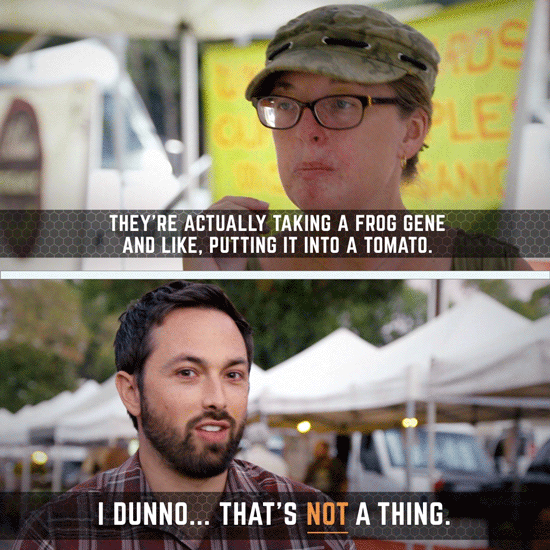
Derek from Veritasium
As of this writing, there are no GM plants on the market whose transgenes were derived from animals, but that is tangential to the even bigger flaw and this line of thinking. The argument is based on the assumption that the number and/or identity of genes altered by a process makes less of a difference than the source of the single gene.
However, this is an incorrect way of looking at it, because at least for life on earth, all species are related by common descent, the genetic code is a nearly universal language, and most genes are not species proprietary [10],[11],[12],[13],[14],[15],[16]. There are very few minor exceptions to the genetic code throughout earth’s biosphere [17]. I can cover DNA composition and the genetic code in more detail in another post if there’s sufficient demand. But for now, suffice it to say that there is a well-known and well-understood relationship between certain combinations of nucleotide bases in protein-coding genes and the amino acids for which they correspond when their mRNA transcripts are translated into proteins [15]. Moreover, all species share certain genes with many other species, and although there can be differences in the ways a gene is expressed in two different species (or even two different cell types within the same organism), those differences occur in ways that can be measured and understood, and they don’t involve outright violations of the genetic code itself [18]. That’s just not how it works.
Often the most noticeable differences between two species has more to do with differential expression and regulation of genes they share in common than with dramatic differences in which genes they possess [18]. And although the passing of genes across species boundaries (horizontal gene transfer) is primarily associated with prokaryotes (bacteria and archaea) in nature, it is not entirely unheard of in eukaryotes. [19],[20].
As an interesting point of fact, the genome of cultivated sweet potatoes has been shown to contain agrobacterium T-DNAs, demonstrating them to be a natural transgenic food crop [21]. This is literally the exact same method used in the deliberate engineering of several transgenic crops today: the agrobacterium method [22]. So, even the claim that transgenesis never occurs in nature is false. Whether or not it occurs naturally obviously has no bearing on its safety, but the point is that many of these anti-GMO arguments are flawed on multiple different levels. The term “fractal wrongness” comes to mind.
Unintended Changes are not Impossible
It is important to point out that unintended off-target changes to the genome can and sometimes do occur with all known genetic modification techniques, including both transgenesis as well as more traditional methods not considered “GMO” by usual colloquial standards. This is neither unexpected nor necessarily problematic, but it is also true that a small portion of these off-target changes may turn out to confer undesirable traits in the resulting organism [23]. However, not only are such instances less likely to make it into the food supply with GE than with non-GE for regulatory reasons, but strong evidence suggests that they also occur less frequently with transgenesis than with most techniques considered non-GE.
How do we know this?
This can be tested in a number of different ways. These methods include compositional, genomic, transcriptomic, proteomic, and metabolomic comparisons between a GE line and a closely-related non-GE counterpart. I will explain each of these in turn, but understand that they are not mutually exclusive.
Compositional Equivalence
One of the most common ways to test this is to perform compositional comparisons between a GE crop and a nearly isogenic non-GE counterpart line. In many instances, one or more additional non-GE commercial reference lines will be included. This usually involves replicated field trials placed throughout a growing region from which tissue samples are taken, which are then analyzed for an array of several dozen compounds.
Subsequently, statistical methods are employed in order to determine whether any significant compositional differences exist between the GE and non-GE lines. If this were to turn out to be the case, the analytes in question would then be compared to the range of levels considered to be normal for the particular crop. If levels are not within the normal range of natural variation, scientists then have to determine the biological relevance of these differences within the context of how the crops are typically produced and consumed. Guidelines for these types of compositional field trials have been prescribed by the EFSA [24]. Notice that this does not entail that the transgenic lines need to be precisely identical to their non-GE counterparts. Some variation is expected. The issue is whether or not the differences actually matter [58].
Genomics
Another way of assessing the prevalence of off-target changes in transgenesis is to compare various genetic markers between related transgenic and non-transgenic lines. Generally speaking, whole genome sequencing is rarely ever used for this purpose. Part of the reason for this is because, until recently, whole genome sequencing had been cost prohibitive. Another reason, however, is because genome sequencing alone doesn’t tell us much about how the rates of expression of different genes might be affected by the modification process. Of the thousands (or even tens of thousands) of genes possessed by a given multi-cellular organism, only a small fraction of them are expressed in any particular cell at any given time [18].
In fact, the phenomenon of differential gene expression between different cell and tissue types is central to the entire existence and development of complex multi-cellular life. Different cells in different tissues can have the exact same nuclear DNA, yet be vastly different in their appearance and function, simply because they express different subsets of those genes in different cells and/or express them at different rates [18].
This is why genome sequencing alone doesn’t tell us the whole story with respect to examining the changes made (whether intended or not) to a particular cultivar, regardless of the breeding method used to accomplish it. For that, it is often more informative to analyze and compare the total set of RNA molecules transcribed in a given set of cells (the transcriptome), and/or the proteins into which some of those RNA molecules are translated (the proteome). More on that in a moment.
Transcriptomics
Recall that when DNA in Eukaryotic cells is transcribed the initial product is an RNA strand called a primary transcript. In the case of protein-coding genes, those primary transcripts are called pre-mRNA (pre-messenger RNA), and typically undergo processing in order to produce mature RNA transcripts, which will later be translated into proteins which perform particular functions within the cell [25]. Not all DNA is transcribed to RNA, and not all RNA is translated into proteins, and not all protein-coding genes are expressed in all cell types [18].
As if that wasn’t already enough reason to conclude that the genome itself doesn’t convey the entire expressive picture, some RNA even has to undergo splicing and tagging in the cell before becoming a mature mRNA transcript ready to be translated into a protein. Some of these transcripts even have alternative splicing patterns. These splice jobs are performed by protein-RNA macromolecular complexes known as small nuclear ribonucleoproteins, or simply snRNPs (pronounced snurps). Their purpose is to splice out sections of the primary transcript called introns, which are not expressed in the final product, thus leaving only regions called exons, which are expressed [25].
Much as the aggregate of an organism’s genes is called its genome, the set of all RNA transcripts within a given cell or tissue of a given organism at a given time is called its transcriptome. Analyzing the transcriptomes of GE organisms and comparing them to those of closely-related organisms altered via other methods gives us an idea of how those modifications are really affecting gene expression.
Other “Omics” Comparisons
If genomics involves analyzing all the genes in an organism’s genome, and transcriptomics involves analyzing all the RNA transcripts of a particular cell at a particular time, then you can probably infer that proteomics refers to the analysis of all the proteins expressed in a particular cell at a particular time [26]. Instead of looking at individual proteins, proteomics looks at all of the proteins present. Once the individual proteins have been identified, researchers can then study how the proteins present change over time, how they interact, and/or how they vary between different cells. Because proteins facilitate so many cellular processes, the proteome serves as a functional representation of the genome.
Similarly, metabolomics is the study of all the metabolites present in a cell, tissue, or organism [27]. These metabolites include the small molecular end products and intermediates of various cellular processes, as well as hormones and some signaling molecules. In human metabolomics, molecules may be sub-classified as either endogenous or exogenous depending on whether or not they’re produced by the organism, or as xenometabolites in the case of foreign substances such as drugs [28],[29]. For plants, the terms primary and secondary metabolites are often used [30]. The former refers to metabolites involved in normal growth, development, and/or reproduction, whereas the latter are not, but will in many cases serve an ecological function of interest.
Like the transcriptome and proteome, the metabolome is dynamic; it changes over time. Consequently, transcriptomics, proteomics, and metabolomics are all important tools comprising the field known as functional genomics. A genome sequence is ostensibly just a parts list, whereas these tools permit insight into what the genome is actually doing. Insofar as applying these techniques to compare the rates of off-target changes of different methods of modification, one of the conclusions of Metzdorf et al 2006 was that correct interpretations of the ‘omics’ data would require information about the range of natural variability of crop plants under examination [31].
GE and Unintended Off-Target Changes: What Does the Evidence Actually Suggest?
The transcriptomics, proteomics, and metabolomics, along with compositional and genomic analyses permit us to compare the rates of unintended off-target changes in GE organisms vs those arrived at via other breeding methods. Indeed, such measures have been used to test exactly that question. The evidence suggests that they involve LESS change. That doesn’t mean a harmful off target change is literally physically impossible, but it does make it less likely than with non-GMO. Here are some examples:
This study analyzed the transcriptome of drought-tolerant transgenic arabidopsis thaliana crops via the use of microarrays. (More on those later). With the exception of the transgene itself (ABF3), which codes for a regulatory transcription factor involved in triggering the expression of other genes associated with drought response, the transcriptome revealed no significant differences in gene expression between the transgenic lines vs the controls [32].
Here’s one which found that transgenesis resulted in fewer off-target changes to the transcriptome of wheat grain than did conventional cross-breeding [33].
The following meta-analysis incorporated studies with multiple-”omics” comparisons. Ricroch et al found that that genetic engineering had less of an impact on plant gene expression and composition relative to conventional plant breeding. They also found that environmental factors such as field location, sampling time, (or agricultural practices) had more impact on outcomes than transgenesis [34].
This metabolomic analysis of transgenic wheat did reveal some subtle differences in amino acid profile as well as maltose and sucrose concentrations relative to conventional parental controls. However, these differences were well within the expected range of natural variation and were dwarfed by differences attributable to location, soil, weather etc [35].
The following excerpt is from a paper looking back on 20 years and over 80 studies of compositional comparison research [36]:
“It is concluded that suspect unintended compositional effects that could be caused by genetic modification have not materialized on the basis of this substantial literature.”
The authors also concluded the following:
“Our assessment is that there appears to be overwhelming evidence that transgenesis is less disruptive of crop composition compared with traditional breeding, which itself has a tremendous history of safety.”
The following proteomic study compared transgenic insect-resistant Bt cotton with conventional using an Enzyme Linked Immunosorbent Assay (ELISA). The author’s found some subtle differences in the expression and/or interaction of 35 proteins, most of which were involved in photosynthetic pathways and/or carbohydrate transport and metabolism, as well as some chaperone proteins involved in post-translational modification. However, there was no increase in toxic or allergenic proteins, and the authors concluded that the differences were minor enough not to constitute a sharp change to the proteome in transgenic cotton leaves [37].
This one found that gene expression differences between insect resistant maize and conventional were best explained by natural variation and environmental considerations [38].
Transgenesis has also been compared with Marker Assisted Breeding, which is a form of selective back crossing for which certain genetic markers are used to ascertain whether or not a plant possesses the desired trait, so that it is not necessary to wait until the plant is fully developed [52]. This speeds up the process. Using transcriptome comparisons between the transgenic and MAB rice cultivars, the authors found that the transgenic rice had a whopping 40% fewer changes in gene expression than the rice arrived at via Marker Assisted Backcrossing relative to the controls [53].
Here is one in which comprehensive metabolomic comparisons were performed on transgenic potatoes relative to closely related cultivars. Other than already anticipated metabolites, the authors’ mass spectra and chromatographic analysis found no significant metabolomic differences between the transgenic lines vs the conventional [39]. These are only but a handful of the studies of these types that are out there. Here are some more [54],[55],[56],[57].
Mutagenesis
Mutagenesis is a common method of modification in which ionizing radiation or mutagenic chemicals are used to speed up the mutation rate of seeds so that desirable traits which randomly emerge can be artificially selected for in subsequent generations [40]. By US standards, plants developed in this way aren’t legally considered “GMO.” Unlike genetically engineered seeds, they can be used in organic farming.
Given that the procedure’s entire purpose is to speed up mutations, it’s reasonable to wonder how their rates of off-target changes compare to those attributable to transgenic techniques.
The following study found that transgenesis resulted in an entire order of magnitude fewer off-target structural variations to the genome relative to mutagenesis. Using what’s called a tilling microarray, the authors also found that, although rare, structural variants in transgenic varieties typically occurred directly adjacent to the points of transgene insertion, or occasionally at unlinked loci on different chromosomes [41]. Note: I had initially included a section in this post describing the premise of DNA microarrays, RNA-seq, but omitted them because they would make the article way too long.
“On average, the number of genes affected by structural variations in transgenic
plants was one order of magnitude less than that of fast neutron mutants and
two orders of magnitude less than the rates observed between cultivars.”
Another study found that transcriptome alteration was greater in mutagenic breeding than with transgenesis [51].
Despite this, seeds arrived at via mutagenesis undergo no safety evaluation or substantial equivalence testing whatsoever prior to commercialization. A coherent justification for this regulatory double standard has not been forthcoming.
Harmful and/or Undesired Results From Conventional Breeding
Regardless of whether they were genetically engineered, all foods contain substances that could be potentially hazardous in sufficiently high amounts. Unlike GE, however, non-GE methods of modification actually have resulted in foods with harmful unintended changes making it into the food supply.
For example, the kiwi fruit, which was brought about by conventional breeding, has been implicated in severe anaphylaxis in predisposed individuals [42].
Similarly, the conventionally bred Lenape potato was found to contain dangerously elevated solanine levels in the late 1960s, and was subsequently removed from the market [43],[44]. Ironically, there have been experiments in which a transgenic version of the Lenape potato were shown to express substantially lower solanine levels [45].
More recently, a similar case arose with a heritage variety of potato developed in the UK, and was subsequently removed from the market [46].
Elevated psoralens levels in celery plants is another example in which high levels of a toxic compound resulted from traditional breeding [47]. These chemicals confer natural resistance to insect predation and make the plant more aesthetically appealing to consumers, but they are also irritants which can become toxic in higher amounts [48].
Cucurbitacins are a class of biochemical compounds produced by some plants as a defense against herbivores [49]. There have been cases in which conventionally bred zucchini squash varieties have produced such compounds in potentially dangerous quantities [50].
To be clear, there do also exist cases in which GE resulted in unintended off-target changes. However, as the comprehensive 2016 literature review by the National Academy of Sciences puts it:
“Because GE crops are regulated to a greater degree than are conventionally bred,
non-GE crops, it is more likely that traits with potentially hazardous characteristics will not pass early developmental phases. For the same reason, it is also more likely that unintentional, potentially hazardous changes will be noticed before commercialization either by the breeding institution or by governmental regulatory agencies.”
Conclusion
The bottom line is that we humans have been modifying our food by one method or another for thousands of years. I am well aware that contrarians to the mainstream scientific position like to over-emphasize that GE is different, and strictly speaking, it is trivially true that GE is different, since every method of genetic alteration is technically (by definition) different than every other method of modification; wide-cross hybridization is different from transgenesis, which is different from protoplast fusion, which is different from miRNA gene silencing, which is different from mutagenesis, which is different from polyploidy, and so on and so forth.
However, that argument is ultimately a red herring, because it ignores that the ways in which GE is different are its strengths: not its weaknesses. Overwhelming scientific evidence indicates that commercially available GE foods are at least as safe as their conventional counterparts, and that the processes itself does not increase the likelihood of harmful unintended off-target changes relative to traditional methods of modification. The latter conclusion is supported by multiple converging lines of genomic, transcriptomic, proteomic, and metabolomic evidence and compositional equivalence studies. Moreover, due to the fact that they are far more stringently regulated, any potentially hazardous unintended changes are far more likely to be caught prior to commercialization with GE foods relative to non-GE.
Furthermore, vague pie-in-the-sky anti-GE arguments invoking potentially harmful future unknowns could be applied to virtually any technology (young or old). Such arguments fail to consider the potential consequences of rejecting the technology, and tend to be incapable of generating testable hypotheses, which consequently renders them unfalsifiable, and therefore unscientific. In the instances where such arguments have been formulated well enough to actually generate testable hypotheses (such as the prediction that GE should result in greater changes to composition and gene expression), they have been demonstrated to be false.
QED.
– Credible Hulk
References:
[1] Hulk, C. (2015). The International Scientific Consensus On Genetically Engineered Food Safety. The Credible Hulk. Retrieved 23 October 2017, from http://crediblehulk.org/index.php/2015/11/22/the-international-scientific-consensus-on-genetically-engineered-food-safety/
[2] Funk, C., & Rainie, L. (2015). Public and Scientists’ Views on Science and Society. Pew Research Center: Internet, Science & Tech. Retrieved 23 October 2017, from http://www.pewinternet.org/2015/01/29/public-and-scientists-views-on-science-and-society/
[3] Caudill, E. (2013). Intelligently designed : How creationists built the campaign against evolution. Urbana, Chicago, and Springfield: University of Illinois Press.
[4] Collomb, J. (2014). The Ideology of Climate Change Denial in the United States. European Journal Of American Studies, 9(1). http://dx.doi.org/10.4000/ejas.10305
[5] Rubin, J., & Rubin, J. (2017). Opinion | Trump’s climate-change denial rattles U.S. businesses. Washington Post. Retrieved 23 October 2017, from https://www.washingtonpost.com/blogs/right-turn/wp/2017/05/09/trumps-climate-change-denial-rattles-u-s-businesses/?utm_term=.487eaafee294
[6] C., L., S., N., W., P., . . . R. (2016). Consensus on consensus: A synthesis of consensus estimates on human-caused global warming. Environmental Research Letters, 11(4). doi:10.1088/1748-9326/11/4/048002
[7] Ruishalme, I. (2013). Is There a Consensus about Climate Change?. Thoughtscapism. Retrieved 23 October 2017, from https://thoughtscapism.com/2015/03/13/is-there-a-consensus-about-climate-change/
[8] Tafuri, Silvio & Martinelli, D & Prato, R & Germinario, C. (2011). [From the struggle for freedom to the denial of evidence: history of the anti-vaccination movements in Europe]. Annali di igiene : medicina preventiva e di comunità. 23. 93-9.
[9] History of Anti-vaccination Movements | History of Vaccines. (2017). Historyofvaccines.org. Retrieved 24 October 2017, from https://www.historyofvaccines.org/content/articles/history-anti-vaccination-movements
[10] Theobald, D. (2010). A formal test of the theory of universal common ancestry. Nature, 465(7295), 219-222. http://dx.doi.org/10.1038/nature09014
[11] Steel, M., & Penny, D. (2010). Origins of life: Common ancestry put to the test. Nature, 465(7295), 168-169. http://dx.doi.org/10.1038/465168a
[12] Weiss, M., Sousa, F., Mrnjavac, N., Neukirchen, S., Roettger, M., Nelson-Sathi, S., & Martin, W. (2016). The physiology and habitat of the last universal common ancestor. Nature Microbiology, 1(9), 16116. http://dx.doi.org/10.1038/nmicrobiol.2016.116
[13] Watanabe, K., & Suzuki, T. (2008). Universal Genetic Code and its Natural Variations. Encyclopedia Of Life Sciences. http://dx.doi.org/10.1002/9780470015902.a0000810.pub2
[14] Koonin, E., & Novozhilov, A. (2009). Origin and evolution of the genetic code: The universal enigma. IUBMB Life, 61(2), 99-111. http://dx.doi.org/10.1002/iub.146
[15] Isenbarger, T., Carr, C., Johnson, S., Finney, M., Church, G., & Gilbert, W. et al. (2008). The Most Conserved Genome Segments for Life Detection on Earth and Other Planets. Origins Of Life And Evolution Of Biospheres, 38(6), 517-533. http://dx.doi.org/10.1007/s11084-008-9148-z
[16] Bejerano, G. (2004). Ultraconserved Elements in the Human Genome. Science, 304(5675), 1321-1325. http://dx.doi.org/10.1126/science.1098119
[17] Turanov, A., Lobanov, A., Fomenko, D., Morrison, H., Sogin, M., & Klobutcher, L. et al. (2009). Genetic Code Supports Targeted Insertion of Two Amino Acids by One Codon. Science, 323(5911), 259-261. http://dx.doi.org/10.1126/science.1164748
[18] Gilbert, S., & Barresi, M. (2016). Developmental biology (6th ed.). Sunderland (Mass.): Sinauer.
[19] Fuentes, I., Stegemann, S., Golczyk, H., Karcher, D., & Bock, R. (2014). Horizontal genome transfer as an asexual path to the formation of new species. Nature, 511(7508), 232-235. http://dx.doi.org/10.1038/nature13291
[20] Marine Biological Laboratory. (2015, February 3). Sea slug has taken genes from algae it eats, allowing it to photosynthesize like a plant. ScienceDaily. Retrieved October 23, 2017 from www.sciencedaily.com/releases/2015/02/150203155925.htm
[21] Kyndt, T., Quispe, D., Zhai, H., Jarret, R., Ghislain, M., & Liu, Q. et al. (2015). The genome of cultivated sweet potato contains AgrobacteriumT-DNAs with expressed genes: An example of a naturally transgenic food crop. Proceedings Of The National Academy Of Sciences, 112(18), 5844-5849. http://dx.doi.org/10.1073/pnas.1419685112
[22] Gelvin, S. (2003). Agrobacterium-Mediated Plant Transformation: the Biology behind the “Gene-Jockeying” Tool. Microbiology And Molecular Biology Reviews, 67(1), 16-37. http://dx.doi.org/10.1128/mmbr.67.1.16-37.2003
[23] Committee on Identifying and Assessing Unintended Effects of Genetically Engineered Foods on Human Health. (2004). Safety of Genetically Engineered Foods (p. 49). Washington: National Academies Press.
[24] EFSA Panel on Genetically Modified Organisms (GMO); Scientific Opinion on Guidance for risk assessment of food and feed from genetically modified plants. EFSA Journal 2011;9(5): 2150. [37 pp.] doi:10.2903/j.efsa.2011.2150.
[25] Mithieux, S., & Weiss, A. (2005). Elastin. Fibrous Proteins: Coiled-Coils, Collagen And Elastomers, 437-461. http://dx.doi.org/10.1016/s0065-3233(05)70013-9
[26] NatureEducation. (2017). Scitable. Nature.com. Retrieved 24 October 2017, from https://www.nature.com/scitable/definition/proteome-297
[27] Griffin, J., & Vidal-Puig, A. (2008). Current challenges in metabolomics for diabetes research: a vital functional genomic tool or just a ploy for gaining funding?. Physiological Genomics, 34(1), 1-5. http://dx.doi.org/10.1152/physiolgenomics.00009.2008
[28] Nordström, A., O’Maille, G., Qin, C., & Siuzdak, G. (2006). Nonlinear Data Alignment for UPLC−MS and HPLC−MS Based Metabolomics: Quantitative Analysis of Endogenous and Exogenous Metabolites in Human Serum. Analytical Chemistry, 78(10), 3289-3295. http://dx.doi.org/10.1021/ac060245f
[29] Crockford, D., Maher, A., Ahmadi, K., Barrett, A., Plumb, R., Wilson, I., & Nicholson, J. (2008). 1H NMR and UPLC-MSE Statistical Heterospectroscopy: Characterization of Drug Metabolites (Xenometabolome) in Epidemiological Studies. Analytical Chemistry, 80(18), 6835-6844. http://dx.doi.org/10.1021/ac801075m
[30] Bentley, R. (1999). Secondary Metabolite Biosynthesis: The First Century. Critical Reviews In Biotechnology, 19(1), 1-40. http://dx.doi.org/10.1080/0738-859991229189
[31] Metzdorff, S., Kok, E., Knuthsen, P., & Pedersen, J. (2006). Evaluation of a non-Targeted “Omic” approach in the safety assessment of genetically modified plants. Plant Biol (stuttg), 8(5), 662-672. doi:10.1055/s-2006-924151
[32] Abdeen, A., Schnell, J., & Miki, B. (2010). Transcriptome analysis reveals absence of unintended effects in drought-tolerant transgenic plants overexpressing the transcription factor ABF3. BMC Genomics, 11(1), 69. http://dx.doi.org/10.1186/1471-2164-11-69
[33] Baudo, M., Lyons, R., Powers, S., Pastori, G., Edwards, K., Holdsworth, M., & Shewry, P. (2006). Transgenesis has less impact on the transcriptome of wheat grain than conventional breeding. Plant Biotechnology Journal, 4(4), 369-380. http://dx.doi.org/10.1111/j.1467-7652.2006.00193.x
[34] Ricroch, A. (2013). Assessment of GE food safety using ‘-omics’ techniques and long-term animal feeding studies. New Biotechnology, 30(4), 349-354. http://dx.doi.org/10.1016/j.nbt.2012.12.001
[35] Herman, R. A., & Price, W. D. (2013). Unintended compositional changes in genetically modified (GM) crops: 20 years of research. Journal of agricultural and food chemistry, 61(48), 11695-11701.
[36] Anderson, J., Michno, J., Kono, T., Stec, A., Campbell, B., Curtin, S., & Stupar, R. (2016). Genomic variation and DNA repair associated with soybean transgenesis: a comparison to cultivars and mutagenized plants. BMC Biotechnology, 16(1). http://dx.doi.org/10.1186/s12896-016-0271-z
[37]. Wang, L., Wang, X., Jin, X., Jia, R., Huang, Q., Tan, Y., & Guo, A. (2015). Comparative proteomics of Bt-transgenic and non-transgenic cotton leaves. Proteome Science, 13(1). http://dx.doi.org/10.1186/s12953-015-0071-8
[38] Coll, A., Nadal, A., Collado, R., Capellades, G., Kubista, M., Messeguer, J., & Pla, M. (2010). Natural variation explains most transcriptomic changes among maize plants of MON810 and comparable non-GM varieties subjected to two N-fertilization farming practices. Plant Molecular Biology, 73(3), 349-362. http://dx.doi.org/10.1007/s11103-010-9624-5
[39]. Catchpole, G., Beckmann, M., Enot, D., Mondhe, M., Zywicki, B., & Taylor, J. et al. (2005). Hierarchical metabolomics demonstrates substantial compositional similarity between genetically modified and conventional potato crops. Proceedings Of The National Academy Of Sciences, 102(40), 14458-14462. http://dx.doi.org/10.1073/pnas.0503955102
[40] Brown, N. (2011). 20. Mutagenesis – PlantBreeding. Plantbreeding.coe.uga.edu. Retrieved 24 October 2017, from http://plantbreeding.coe.uga.edu/index.php?title=20._Mutagenesis
[41] Anderson, J., Michno, J., Kono, T., Stec, A., Campbell, B., Curtin, S., & Stupar, R. (2016). Genomic variation and DNA repair associated with soybean transgenesis: a comparison to cultivars and mutagenized plants. BMC Biotechnology, 16(1). http://dx.doi.org/10.1186/s12896-016-0271-z
[42]. K., S., R., O., & M. (2007). Life-threatening anaphylaxis to kiwi fruit: Protective sublingual allergen immunotherapy effect persists even after discontinuation. The Journal of Allergy and Clinical Immunology, 119(2), 507-8.
[43] Akeley, R., Mills, W., Cunningham, C., & Watts, J. (1968). Lenape: A new potato variety high in solids and chipping quality. American Potato Journal, 45(4), 142-145. http://dx.doi.org/10.1007/bf02863068
[44] Zitnak, A., & Johnston, G. (1970). Glycoalkaloid content of B5141-6 potatoes. American Potato Journal, 47(7), 256-260. http://dx.doi.org/10.1007/bf02864825
[45] McCue, K., Allen, P., Rockhold, D., Maccree, M., Belknap, W., & Shephard, L. et al. (2003). Reduction of Total Steroidal Glycoalkaloids in Potato Tubers Using Antisense Constructs of a Gene Encoding A Solanidine Glucosyl Transferase. Acta Horticulturae, (619), 77-86. http://dx.doi.org/10.17660/actahortic.2003.619.9
[46] Hellenäs, K., Branzell, C., Johnsson, H., & Slanina, P. (1995). High levels of glycoalkaloids in the established swedish potato variety magnum bonum. Journal Of The Science Of Food And Agriculture, 68(2), 249-255. http://dx.doi.org/10.1002/jsfa.2740680217
[47] Beier, R. (1990). Natural Pesticides and Bioactive Components in Foods. Reviews Of Environmental Contamination And Toxicology, 47-137. http://dx.doi.org/10.1007/978-1-4612-3366-4_2
[48] Beier, R., & Oertli, E. (1983). Psoralen and other linear furocoumarins as phytoalexins in celery. Phytochemistry, 22(11), 2595-2597. http://dx.doi.org/10.1016/0031-9422(83)80173-3
[49] Ferguson, J., Fischer, D., & Metcalf, R. (1983). A report of cucurbitacin poisonings in humans. Cgc, V.6, P.73-74, 1983.
[50] Rymal, K., Chambliss, O., Bond, M., & Smith, D. (1984). Squash containing toxic cucurbitacin compounds occurring in california and alabama. Journal of Food Protection, 47(4), 270-271. doi:10.4315/0362-028X-47.4.270
[51] Batista, R., Saibo, N., Lourenço, T., & Oliveira, M. M. (2008). Microarray analyses reveal that plant mutagenesis may induce more transcriptomic changes than transgene insertion. Proceedings of the National Academy of Sciences, 105(9), 3640-3645.T
[52] Collard, B. C. Y., Jahufer, M. Z. Z., Brouwer, J. B., & Pang, E. C. K. (2005). An introduction to markers, quantitative trait loci (QTL) mapping and marker-assisted selection for crop improvement: the basic concepts. Euphytica, 142(1-2), 169-196.
[53] Gao, L., Cao, Y., Xia, Z., Jiang, G., Liu, G., Zhang, W., & Zhai, W. (2013). Do transgenesis and marker-assisted backcross breeding produce substantially equivalent plants?-A comparative study of transgenic and backcross rice carrying bacterial blight resistant gene Xa21. BMC genomics, 14(1), 738.
[54] Baudo, M. M., Lyons, R., Powers, S., Pastori, G. M., Edwards, K. J., Holdsworth, M. J., & Shewry, P. R. (2006). Transgenesis has less impact on the transcriptome of wheat grain than conventional breeding. Plant Biotechnology Journal, 4(4), 369-380.
[55] Gregersen, P. L., Brinch-Pedersen, H., & Holm, P. B. (2005). A microarray-based comparative analysis of gene expression profiles during grain development in transgenic and wild type wheat. Transgenic research, 14(6), 887-905.
[56] Coll, A., Nadal, A., Palaudelmas, M., Messeguer, J., Melé, E., Puigdomenech, P., & Pla, M. (2008). Lack of repeatable differential expression patterns between MON810 and comparable commercial varieties of maize. Plant molecular biology, 68(1-2), 105-117.
[57] Harrigan, G. G., Lundry, D., Drury, S., Berman, K., Riordan, S. G., Nemeth, M. A., … & Glenn, K. C. (2010). Natural variation in crop composition and the impact of transgenesis. Nature biotechnology, 28(5), 402-404.
3 Comments
R R Deehan · November 13, 2017 at 6:13 pm
Hulk, I wonder whether I could trouble you for some help on a definition I need. I’ve looked for a ‘contact the Hulk’ page, but failed to find one, hence this comment 🙂
What I basically need is a *really* simple explanation of what the laws of physics are, and I’m struggling. So far, all I’ve managed is this:-
the laws of physics are ultimately just our current best descriptions of the state a bit of the universe needs to be in (the ’cause’) in order for another state (the ‘effect’) to arise consistently. Those laws are built from observations, and multiple observations allow us to whittle away everything that isn’t absolutely necessary, leaving the simplest description of the circumstances that cause the effect. We can even infer very general models, that describe causality across a vast range of circumstances. The motion of a ball-bearing falling from your hand and the motion of an asteroid being pulled into the sun can be described using essentially the same model, for example. It’s not a foolproof method, and although they are called ‘laws’ there’s no guarantee they won’t at some point be replaced by even better versions, in the same way that Newton’s model of gravity gives way to Einstein’s. Although they aren’t perfect, these generalised descriptions of causality can model reality well enough to enable us to manipulate the universe to an incredible extent by exploiting those consistent causal relationships, and can even enable us to predict when an event will happen again, which is the main reason why you are surrounded by our amazing technological civilisation. Every time you play ‘Pokemon’ on your iPhone you demonstrate the accuracy of our physical laws, you could say.
If you can help me improve on it or point me towards something better, I’d really appreciate it. Great FB page, BTW. Always cheers me up to read your latest smackdown on the brainfarks. I’ll understand, of course, if you are too busy to help.
Keep on smashing, big guy!
R R Deehan
Credible Hulk · January 9, 2018 at 2:42 am
It’s useful to make clear the distinction between facts, laws and theories in science. I covered this in my article about the correspondence principle, which deals with how certain aspects of older laws and theories are preserved in modified form and become incorporated into new and improved ones. Yes, it’s true that we can never rule out the possibility that we may come across an even better model or explanation for how something works, but the predictions of an older model or theory which made successfully place constraints on the ways in which scientific ideas can change and evolve with new evidence.
http://crediblehulk.org/index.php/2018/01/05/incommensurability-the-correspondence-principle-and-the-scientists-were-wrong-before-gambit/
R R Deehan · January 10, 2018 at 9:31 am
Thanks Hulk. In the intervening time, I refined the passage to the point where I’m happy with it, and my cat can understand it. I’ll have a read of your post anyways – no substitute for maximum input, as they say. Happy New Year, BTW.
Comments are closed.